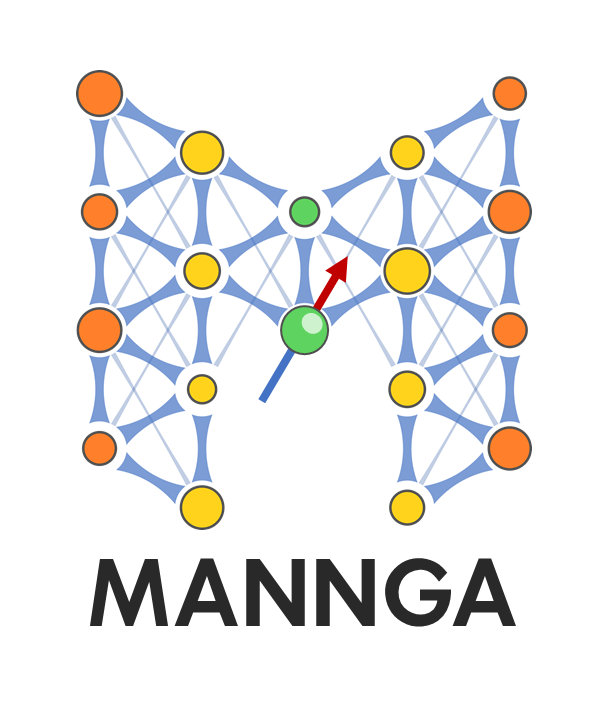
Work package descriptions
Work package 1 Management and coordination
Objectives
- To manage the contract and to keep the EC informed about the current and overall progress achieved in MANNGA.
- To ensure an efficient transfer of knowledge generated in the course of the project within the consortium and to the wider scientific community.
Work package 2 Individual chiral magnonic resonators
Objectives
To lay the groundwork for later stages of the project by implementing, characterising, and optimising 2D chiral magnonic resonators as
- sources of coherent spin waves;
- control elements for chiral, incidence angle-dependent spin-wave amplitude transmission and phase-inversion;
- output couplers for spin-wave detection.
This work package encompasses activities related to design, fabrication, and dynamical characterisation and testing of individual chiral magnonic resonators as building blocks of the more complex magnonic devices to be developed in the subsequent work packages.
SEM images of freestanding 3D YIG resonators
[F. Heyroth, et al, Monocrystalline Freestanding 3D YIG Magnon Nanoresonators, Phys. Rev. Appl. 12, 054031 (2019)].Nominal YIG thickness is 210 nm. The right image also shows a standing spin-wave profile measured by time resolved scanning Kerr microscopy (TRSKM).
Spin-wave control using Fabry-Perot chiral magnonic resonators
[H. Qin, et al, Nanoscale magnonic Fabry-Pérot resonator for low-loss SW control, Nature Communications 12, 2293 (2021)].(a) A magnetic (FM) stripe above a low-loss YIG film. (b) Spin-wave dispersion for an uncovered YIG (λ1) and a YIG (100 nm)/CoFeB (50 nm) bilayer with a 5 nm spacer: the dipolar coupling between the FM and YIG layers leads to an asymmetric spin-wave dispersion. (c) Measured spin-wave transmission spectra of the structure in (b) with (blue) and without (orange) the chiral magnonic resonators of 6 µm and 270 nm widths: the resonator’s edges scatter the spin waves; destructive interference between spin waves entering and circulating the resonator’s leads to discrete gaps in the transmission spectrum. The spin-wave wavelength is shortened in the chiral magnonic resonator, enabling its miniaturisation: the chiral magnonic resonator is ~37 times smaller than the incident spin-wave wavelength in the case shown. The resonator’s magnetisation controls the spin-wave transmission.
Work package 3 Chiral magnonic logic gates and field programmable gate arrays
Objectives
- To implement, characterise, and optimise chiral magnonic logic gates and field programmable gate arrays.
In this work package, we will combine several chiral magnonic resonators on a single chip so as to create magnonic logic gates and field programmable gate arrays. Different programmability strategies will be developed and evaluated in terms of the required field, current, optical or microwave power, speed, reliability, repeatability etc. We will target the devices to perform as NAND and / or XNOR gates (“universal gates”), and / or as a full adder.
The generic magnonic device implemented using chiral magnonic resonators.
[V. V. Kruglyak, Chiral Magnonic Resonators: Rediscovering the Basic Magnetic Chirality in Magnonics, Appl. Phys. Lett. 119, 200502 (2021)]The uniform modes in the input and output resonators are inductively coupled to the external microwave circuitry (not shown). The dark mode in the wider control resonator does not couple to the external microwave fields but can affect the phase and amplitude of spin waves propagating in the magnonic medium.
Work package 4 Magnonic reservoir computers and recurrent neural networks
Objectives
- To implement, characterise, and optimise magnonic reservoir computers and neural networks.
In this work package, we will use expertise and (where possible) data and devices from the previous work packages to explore magnonic reservoir computers and recurrent neural networks. In particular, we will explore various feedback and training mechanisms and will apply our devices to common benchmarking tasks, established in machine learning.
Visionary green magnonic chip: a wirelessly driven array of chiral magnonics resonators acting as a magnonic artificial neural network.
[V. V. Kruglyak, Chiral Magnonic Resonators: Rediscovering the Basic Magnetic Chirality in Magnonics, Appl. Phys. Lett. 119, 200502 (2021)]Chiral magnonic resonators, formed on a low loss YIG magnonic medium, are designed to serve as “neurons” in the input , hidden , and output layers. The arrows show connections enabled by propagating spin waves. Magnetic states defining properties of , and can all be used to programme or train the chip, depending on the chip’s intended function.
Work package 5 Above and beyond
Objectives
- To take advantage of collaboration with other projects funded through the Advanced Spintronics call.
- To advance YIG technology for studies in magnonics and beyond.
- To explore additional opportunities whose benefit for MANNGA’s main objectives is not yet established.
This work package encompasses additional research activities that will allow the consortium to go above and beyond its main commitments.
Work package 6 Benchmarking and technology readiness level assessment
Objectives
- To consolidate the methodology of KPI assessment of magnonic devices created in MANNGA and beyond.
- To track the technology readiness level achieved by the consortium.
- To steer MANNGA’s progress towards realising its full applied potential.
This work package encompasses activities aiming at observing and steering our progress towards applications, while evaluating commercial value of the generated intellectual property.